Towering redwoods. Majestic mountain peaks. Complex cloud formations. As we take moments to appreciate nature’s large displays of beauty, let us recognize the small engineering feats that allow us to live comfortably.
The study of microfluidics contributes significantly to many fields affecting our daily lives.
From monitoring blood glucose to detecting pathogens, microfluidic devices carry tiny amounts of fluid to give accurate test results to caregivers and patients.
Having helped develop and produce multiple microfluidic devices, Strouse has had the opportunity to become involved in and familiar with this niche yet increasingly prominent scientific industry.
If you’re interested in learning more about what microfluidics is or how it works with examples, the following should boost your confidence by answering your questions.
What Is Microfluidics?
Microfluidics is the science of fluids on the micro and nanometer scale.
How small is that? Well, 1 millimeter equals 1,000 micrometers, so microfluidic work is done on a scale of about 1/1,000th of a millimeter. For reference, typical bacteria is 0.2 to 2.0 micrometers in diameter.
Engineers and scientists refer to microfluidics as a subcategory of fluid mechanics. However, it runs on a much smaller scale than regular fluid mechanics.
Microfluidics provides the ability to manipulate small volumes of fluid very precisely, allowing for the preparation of small biological (or synthetic) molecules for testing.
How do Microfluidics Work?
Microfluidics utilizes precise fluid control within small volumes and space to reduce the size of labs and samples. You’re often dealing with volumes of liquid at a measurement of one-millionth of a liter (known as a microliter) or one-billionth of a liter (known as a nanoliter).
Since scientists perform experiments on a tiny scale, they need special tools, such as microfluidic pumps (to supply fluids continuously) and microfluidic valves (to inject precise volumes of samples). The tools and methods of liquid control are highly dependent on sample size because fluid mechanics operate differently on such a small scale.
FLOW DIFFERENCES
Fluid engineers describe how fluid flows in two ways: turbulent and laminar flow. A fluid typically flows in a turbulent manner. When the liquid begins to go through a closed channel, such as a pipe, it swirls around. In the micro-scale, fluid flows parallel instead of swirling around, with particles flowing side by side, or laminar.
Think about a river. Water moving down a river looks turbulent, with currents and eddies evident to the visible eye. On the micro-scale, fluid flows smoothly, with all parts running parallel, so we don’t typically see turbulent flow on the micro-scale.
SURFACE TENSION
Surface tension also differs in micro-scale for fluids. The fundamental laws of fluid mechanics still apply to microfluidics; however, engineers and scientists may neglect to factor in the effects of fluid turbulence and gravity on a single droplet of liquid. Instead, scientists use simplified versions of the same equations when working with microfluidic systems.
What is Microfluidics Used For?
Firstly, let’s take a step back to when it began. From the 1950s onward, five primary applications arose or greatly benefitted from microfluidic research:
1) INKJET AND TRANSISTORS (1950-1959)The first transistor resulted from microfluidics and microtechnology. Inkjet printhead technology emerged around the same time scientists developed ways to get tiny tubes to transport ink.
2) MINI COMPUTERS (1960-1969)Engineers miniaturized computers for space exploration, and scientists developed the first integrated circuits and microprocessors. Photolithography led to miniaturizing thousands of transistors on small semiconductor wafers. These technologies led to pressure sensor production.
3) GAS CHROMATOGRAPH (1970-1979)Engineers and scientists developed the first miniaturized gas chromatograph containing mechanical micro-elements integrated on a silicon wafer. Gas chromatographs allow scientists to analyze air pollutants, alcohol in blood, essential oils, and food products.
4) PRESSURE SENSORS AND PRINTHEADS (1980-1989)MEMS (Micro-electromechanical Systems) came out, which led to the development of pressure sensors and printheads. By the decade's end, scientists had developed microvalves and micropumps for microfluidics. Scientists and engineers continued to develop the field by focusing on silicon-based analysis systems instead of glass systems that required heavy industry facilities.
5) CHEMICAL WARFARE AND POLYMERS (1990-1999)The Defence Advanced Research Projects Agency, a military agency, supported microfluidics research because of its usefulness in detecting portable biological and chemical warfare agents. Scientists launched a research area focused on sensor function in a full lab analysis on a single microfluidic chip. Late in the decade, scientists and manufacturers developed and produced cheaper microfluidic devices using polymer moldings rather than silicone.
MODERN APPLICATIONS
At this point, you’re probably thinking, “Okay, cool. But what does modern microfluidics look like?”
The healthcare industry is full of revolutionary, rapidly evolving inventions like diabetic strips and surgical tape. Medical adhesive advancements include blood glucose strips, diagnostics devices, and microfluidic chips (which we’ll explore momentarily).
The cosmetics industry benefits significantly from emulsions and formulations, and doctors have discovered certain drugs in pharmaceuticals because of microfluidics.
Chemists continue developing studies in flow synthesis and stoichiometry. In energy, engineers study enhanced oil recovery (EOR); microfluidics allows engineers to learn how fluid flows and to create more efficient ways to extract oil.
The science behind adhesive solutions has roots in microfluidics, which led to the improvement of simple adhesive materials such as masking tape, complex diabetic test strips, and advanced medical tape.
Many companies produce micropumps, mixers, microvalves, and other devices for microfluidic work, and demand has lowered the cost of microfluidics. With more affordable devices and materials, numerous labs can conduct research for microfluidic applications.
What is a Microfluidic Chip?
Think back to the evolution of computer technology; in the 1960s, a computer took up a room. Now, you can carry even more power in your hand with your smartphone. The optimization of computers mirrors the advancements achieved in microfluidics.
Scientists and engineers describe microfluidics as technology that’s “lab-on-a-chip” (LoC) or an “organ-on-a-chip.” These chips can function on their own or as components within microfluidic devices.
A microfluidic chip has molded and engraved microfluidics channels with several holes of different dimensions where an individual can inject and evacuate the microfluid. Scientists direct, mix, separate, or manipulate the fluids to create effects throughout the systems. Microfluidic chips are precisely designed to achieve stated goals, whether detecting pathogens, analyzing DNA, or many more tasks.
As we know, moving a large channel of fluids requires pumps and valves. Microfluidic chips also need specific systems to manage fluids but in tiny channels. These systems are quake valves and pressure controllers specific to microfluidics.
While we previously covered the critical materials required to build a microfluidic device, here are the working details about the two most common types of chips:
LAB-ON-A-CHIP
Imagine a full-scale lab where all experiments use normal-sized beakers and tubes. There’s a limited work area, right? With lab-on-a-chip, a scientist works on a micro or miniaturized scale, analyzing several experiments simultaneously on a chip.
At this scale, scientists can generate samples on location (rather than moving them to a lab), and easily control the movement and interaction of samples. The reactions are more potent at this scale, and there’s less chemical waste.
Scientists can reduce exposure when working with dangerous chemicals because there’s such a small amount of the chemical. Instead of exposure to a liter of a chemical, they’re only exposed to a drop.
It’s worth noting that the materials within microfluidic devices must be kept clean to achieve the expected performance. That’s done by a converter meeting ISO cleanroom standards and adhering to strict manufacturing guidelines.
ORGAN-ON-A-CHIP
With organ-on-a-chip, a three-dimensional cell culture is developed on a microdevice, which attempts to reproduce a living organ’s major functions, only on a computer chip.
More efficient than traditional cell culture techniques, organ-on-a-chip mimics microenvironments as well as the function of the organ. A scientist can research human physiology for a specific organ while testing advancements for diseases on that model.
Microfluidics and microfabrication technology allow scientists to recreate the functions of a living organism on a small scale. Examples include: heart on a chip, liver on a chip, tumor on a chip, and even multiple organs on one chip.
Why is Microfluidics so Important?
Although invented in the 1950s, microfluidics began taking off in the early 1990s and has grown exponentially. Life science research and biotechnologies, in particular, find advantages in microfluidics for several reasons:
- Size — When working with microfluidics, you’ll require fewer samples to work with to make the same discoveries. Scientists can do experiments on a small scale and not waste samples.
- Time — Because you’re working with small samples, reactions happen more quickly, shortening the time of experiments and providing faster results
- Cost — Microfluidics can cost less because scientists use fewer samples and take less time to complete experiments.
And that’s not to mention the technical benefits of working with microfluidic applications:
- Precision — Scientists improve the precision of experiments because they’re already working with such precise measurements. If they can make things happen at this level, they’ll have precise results and know how to make the same experiment happen accurately on a larger scale.
- Limits — Scientists can detect changes at a much lower limit than when working in a larger setting. This leads to faster detection of pathogens and viruses.
- Multiple Analyses — You’re using less space and material, and as a result, you can conduct several analyses at the same time. In medicine, microfluidics’ analyses of fluids regularly reduce hospital visits and overall healthcare costs.
The Future of Microfluidics
Microfluidics is a rapidly evolving industry, and each new year brings a wealth of innovative research studies and optimized means of production.
Microfluidic applications affect more industries than just healthcare. From the experiments it once was, the study of microfluidics has led to complete labs dedicated to it worldwide.
As someone interested in microfluidics, you might be developing your own design.
If you’re curious about developing a medical product, consider checking out our Learning Center for further insight on designing a product, choosing suitable materials, and discovering the right manufacturer.
Originally published: February 3, 2021
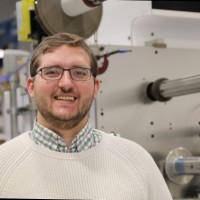